Modeling Titans Circulation With A New GCM
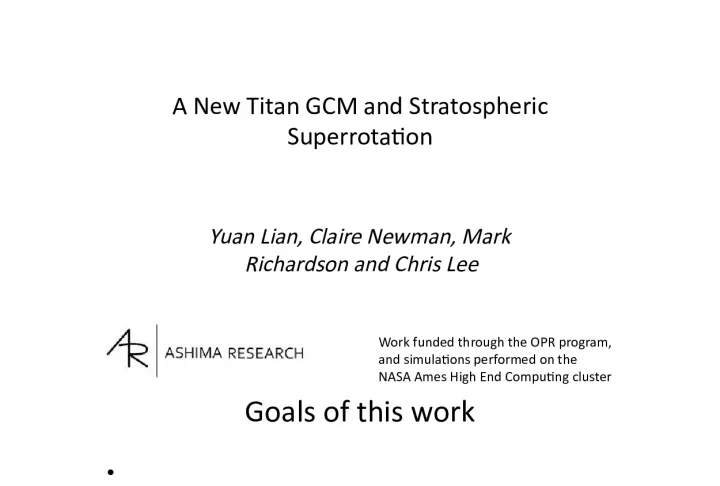
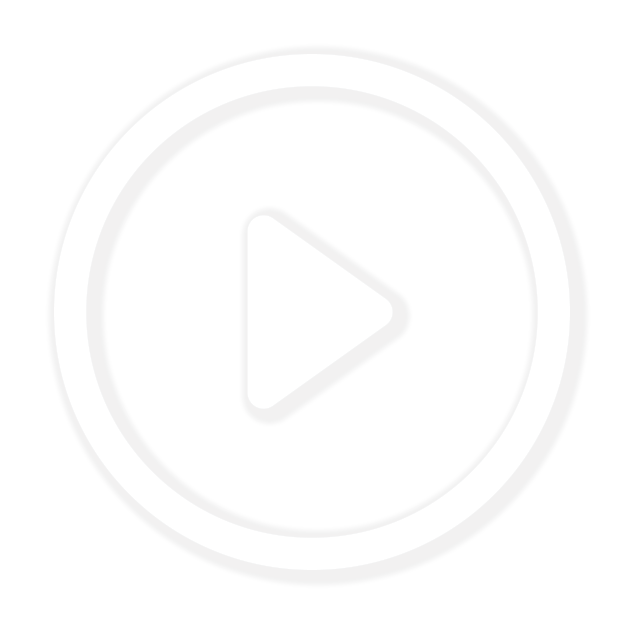
Funded by OPR program, this work aims to understand Titans circulation using a 3D GCM. Simulations on NASA Ames cluster will help predict seasonal changes in circulation and identify dynamical mechanisms behind circulation.
- Uploaded on | 0 Views
-
ninamoore
About Modeling Titans Circulation With A New GCM
PowerPoint presentation about 'Modeling Titans Circulation With A New GCM'. This presentation describes the topic on Funded by OPR program, this work aims to understand Titans circulation using a 3D GCM. Simulations on NASA Ames cluster will help predict seasonal changes in circulation and identify dynamical mechanisms behind circulation.. The key topics included in this slideshow are . Download this presentation absolutely free.
Presentation Transcript
1. A New Titan GCM and Stratospheric Superrotation Yuan Lian, Claire Newman, Mark Richardson and Chris Lee Work funded through the OPR program, and simulations performed on the NASA Ames High End Computing cluster
2. Goals of this work To reproduce key aspects of Titans circulation in a 3-dimensional general circulation model (GCM) To form a robust understanding of the dynamical mechanisms responsible To produce robust predictions of seasonal changes in Titans circulation
3. In previous work, we simulated strong, realistic stratospheric superrotation and seasonal change similar to that observed -- Zonal winds in northern summer Zonal winds in southern summer Pressure (mbar) Comparing the TitanWRF GCM with observations
4. Zonal winds from CIRS in 2005 (Ls~293-323) Zonal winds from CIRS in 2011 (Ls~20- 26) [from Achterberg et al. ] -60 -30 0 30 60 Latitude (deg N) -90 -60 -30 0 30 60 90 Latitude (deg N) 1e -3 1e -2 1e -1 1e 0 1e 1 1e 2 1e 3 1e -3 1e -2 1e -1 1e 0 1e 1 1e 2 1e 3 -90 -60 -30 0 30 60 90 Latitude (deg N) TitanWRF predictions for same times
5. Pressure (mbar) CIRS zonal winds for L s ~293-323 TitanWRF zonal winds for same period Zonal wind peaks at a lower altitude than observed (likely due to the low model top and/or the lack of active haze advection) Discrepancies between TitanWRF and observations Also, no lower stratosphere zonal wind minimum as seen by Huygens and also Cassini (Flasar, 2012)
6. Stratospheric superrotation in TitanWRF We find superrotation is produced by episodic transfer events Planetocentric solar longitude (in L s ) d(angular momentum)/dt in kg m2/s2 Southern dM/dt (Pole to 22.5S) Northern dM/dt (Pole to 22.5N) Equatorial dM/dt (22.5S to 22.5N) Rate of change of angular momentum in 3 regions over a Titan year
7. Stratospheric superrotation in TitanWRF Planetocentric solar longitude (in L s ) Angular momentum transfer events between northern / southern hemisphere and equatorial region , in northern / southern late fall-spring
8. Unstable region develops on low-latitude flank of ~winter zonal jet Waves carry westward angular momentum -> jet => accelerate low latitudes Waves break depositing westward angular momentum => decelerate high latitudes Momentum transport during a transfer event In TitanWRF we found too much atmospheric mixing disrupts these delicate wave processes, leading to weak stratospheric circulations
9. Question 1: Does another GCM using identical radiative forcing produce a similar circulation? - This is actually a well-known problem in Titan modeling Question 2: Do we see episodic transfer events in a different Titan GCM? - How robust is our proposed superrotation mechanism? Question 3: How delicate are the wave interactions involved in driving Titans equatorial superrotation? - Was the need to minimize mixing limited to TitanWRF? Questions we wanted to answer
10. We examined t wo GCMs and four setups. Setups 1 & 2 used our first Titan GCM, TitanWRF 1. T itanWRF [Newman et al ., Icarus, 2011] Lat-lon grid, finite-difference solver 2. TitanWRF with a rotated pole N umerical pole and polar filtering now at the equator Filtering to avoid instabilities where grid spacing is small Grid rotated through 90
11. while setups 3 & 4 used our new second Titan model, the Titan MITgcm 3. Titan M ITgcm [Mars version described in Lian et al ., Icarus, 2012] Lat-lon grid, finite-volume solver 4. Titan MITgcm using cubed- sphere grid No singularities at poles Special points at cube corners
12. Which produced realistic superrotation? 1. T itanWRF with a standard lat-lon grid 2. Titan MITgcm with a standard l at-lon grid Which had problems? 2. TitanWRF with rotated pole 4. MITgcm with cubed-sphere grid
13. What do the problem set-ups have in common? In both cases, w ere messing with the low- to mid- latitudes where the waves are produced that are crucial to driving superrotation TitanWRF with rotated pole Titan MITgcm with cubed-sphere grid Has filtered regions at both numerical poles Has 6 special corner points
14. Superrotation index for the MITgcm lat-lon grid Superrotation index = mass-weighted angular momentum of layer that of same layer at rest wrt the solid surface
15. Superrotation index for the MIT cubed-sphere grid Far weaker superrotation is achieved with the cubed-sphere grid
16. Question 3: How delicate are the wave interactions involved in driving Titans equatorial superrotation? - Was the need to minimize mixing limited to TitanWRF? Answer from this work: The dynamics of the low- to mid-latitudes should be treated very carefully to avoid disrupting vital wave-mean flow interactions - This does not seem to be limited to TitanWRF Questions we wanted to answer
17. Planetocentric solar longitude (in L s ) dM/dt in kg m2/s2 TitanWRF Another year of TitanWRF dM/dt in kg m2/s2
18. Planetocentric solar longitude (in L s ) dM/dt in kg m2/s2 TitanWRF Titan MITgcm dM/dt in kg m2/s2
19. Question 2: Do we see episodic transfer events in a different Titan GCM? - How robust is our proposed superrotation mechanism? Answer from this work: Momentum transport in the Titan MITgcm is remarkably close to that in TitanWRF despite big differences in dynamical core / numerics - The mechanism appears to be quite robust Questions we wanted to answer
20. The circulation in TitanWRF and the MITgcm Comparing winds at L s = 270: TitanWRF has larger peak wind speeds, but Titan MITgcm simulates a strong zonal wind minimum Pressure (mbar) TitanWRF Titan MITgcm
21. The circulation in TitanWRF and the MITgcm Comparing temperatures at L s = 270: largely look very similar, but slight variations can have a big impact on dynamics Pressure (mbar) TitanWRF Titan MITgcm
22. Question 1: Does another GCM using identical radiative forcing produce a similar circulation? - This is actually a well-known problem in Titan modeling Answer: Many similarities, but differences in detail: e.g. superrotation strength; sharper vertical gradients - Much more to investigate here! Questions we wanted to answer
23. Conclusions At Ashima we now have two superrotating Titan GCMs with similarly realistic circulations: TitanWRF and Titan MITgcm Our proposed mechanism for the production of equatorial stratospheric superrotation in TitanWRF via episodic transfer events is supported by Titan MITgcm results As found before, GCM set-ups that disrupt the low- to mid- latitudes ( e.g. too much diffusion, filtering, etc .) disrupt the delicate wave momentum transports responsible Titan MITgcm also captures far more of the observed zonal wind minimum above the tropopause than TitanWRF Could be due to improved accuracy of temperature advection However, tropospheric wind speeds in Titan MITgcm are too small
24. Future work Raise the model top for both models to cover more of the haze production zone Turn on radiatively active advection of haze particles to enable feedbacks between haze distribution, heating and circulation These changes should allow a stronger circulation to develop and reduce interference by the model top, thus improving the match to observations
25. Tropospheric methane ice cloud predicted by TitanWRF More future work: study the CH 4 cycle in the MITgcm!